ALLOMETRIC GROWTH
Allometry is the study of relative growth, of changes in
proportion with increase in size.
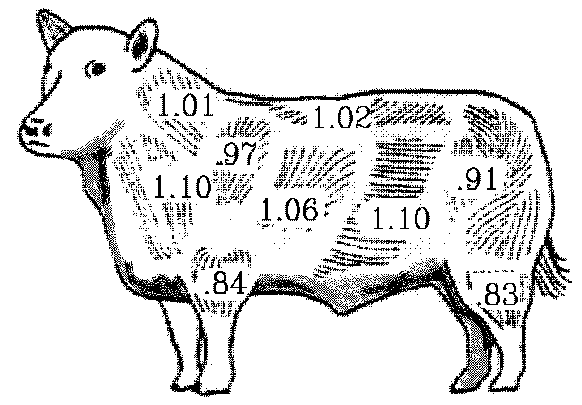
Allometric growth ratios for muscle groups of beef.
- Albrecht Durer (1471-1528), famous German painter and engraver,
used to draw the shapes of animals onto home-made graph paper to
analyse their relative proportions. Nowadays, the cartesian
coordinates may be transformed mathematically and an anatomical
plan stretched to its new shape, thus facilitating the perception
of ontogenetic and phylogenetic changes in shape.
- Before the advent of computer graphics, this technique was too
difficult for the routine analysis of body shape and, without a
computer, the technique is largely subjective, since in most cases
it is easier to fit a transformed cartesian grid to a drawing than
vice versa. Thus, there is a risk of merely using the cartesian
transformation to illustrate a preconceived idea of a shape change.
- Sir John Hammond, one of pioneers in the study of farm animal
growth, simplified the application of this method of analysis by
making photographic prints of animals at magnifications giving a
constant skull length.
- Lateral views of animals at constant skull
length were superimposed onto graph paper grids to demonstrate the
major changes in animal shape resulting from the domestication and
selective breeding of farm animals.
- Thus, when skull length was
held constant photographically, the loins and hams of meat-type
pigs appeared to balloon-out phlyogenetically from the diminutive
rump of the wild boar.
- The same technique revealed a comparable
inflation of the HAM as pigs grew from birth to slaughter
weight.
- At birth, meat animals tend to have large heads and long slender
limbs. Subsequent growth is marked by an increase in body length
and depth until, finally, the hams fill out convexly. Hammond's
technique showed the animal shape that would be most likely to
yield the greatest mass of edible meat.
- Unfortunately, shape then
became a more important criterion than yield, despite the fact that
many generations of blocky meat animals failed to yield their
promised return of lean meat on the butcher's block. The problem
was casued by subcutaneous and intermuscular fat.
To estimate meat
yield in live animals it is necessary to visualize the muscle mass
beneath the animal's outward shape.
- Body regions where subcutaneous
fat is scarce, and where muscle mass may be judged by the stance
between the limbs are, therefore, particularly important in the
judgement of live meat animals. Unfortunately, as cattle grow
fatter, fat deposition tends to shift from relatively inexpensive
cuts in the ventral part of the carcass to the more valuable cuts
of the dorsal part of the carcass.
- Hammond's concept of the ontogeny of shape in sheep was:
- that a
wave of growth starts from the head and passes back along the
vertebral column.
- that secondary waves in the limbs pass from distal to
proximal,
- that the union of the anterior to posterior wave in the
vertebral column, with the distal to proximal wave in the hindlimb
results in the late, but extensive growth of the pelvic region,
- tha the difference in shape between the fetus and adult
resembles the shape change that occurred in the transformation of
wild to domestic farm mammals.
The major changes in both individual development (ONTOGENY) and in evolutionary change (PHYLOGENY) are a reduction in the head relative to the body, and a reduction
in the limbs relative to the trunk of the body. In pigs, however,
the anterior to posterior wave may or may not be detectable.
<
Allometric growth ratio
Sir Julian Huxley in 1932 described a simple mathematical
method for the detection and measurement of the allometric growth.
In order to compare the relative growth of two components (one of
which may be the whole body), they are plotted logarithmically on
X and Y axes,
log y = log b + k log x
The slope of the resulting regression is called the allometric
growth ratio, often designated as k.
- With k = 1, both components are growing at the same rate.
- With k < 1, the component represented on the Y axis is growing
more slowly than the component on the X axis.
- With k > 1, the Y axis component is growing faster than the X
axis component.
This equation has become popular because it appears to work well:
scattered data points are pulled into a neat line with a high
coefficient of correlation. However, these features do not
automatically prove that a logarithmic transformation is
necessarily the best or the only way in which to transform a set of
data.
Allometric growth is normally regarded in a positive light.
Thus, a unit of the body with a positive allometric growth ratio (k
> 1) is thought of as exhibiting rapid relative growth. When we
attempt to fit the phenomenon of allometric growth into the general
framework of our knowledge of sigmoid growth curves, the concept of
allometric growth as a positive phenomenon is inappropriate. During
the period of growth after birth (and even somewhat before birth)
the velocity of growth tends to decline. Thus, those parts of the
body with high postnatal allometric growth ratios also may be
regarded as retarded parts, relative to those parts that have
already decelerated their growth.
Allometric growth of carcass muscles
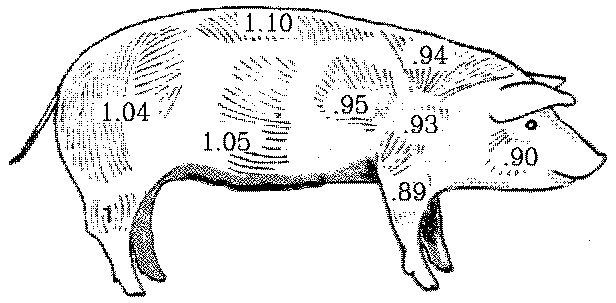
Allometric growth ratios for muscle groups of the pig.
Allometric growth ratio may be used to categorize muscles into one
of three monophasic categories (high, average and low impetus)
depending on whether their allometric growth ratio is greater than,
equal to, or less than a value of 1.
Many of the postnatal allometric growth ratios of muscles change
during development so that a diphasic or dual categorization was
needed.
The dominant diphasic types are:
- high followed by average,
- average followed by high,
- and low followed by average.
The growth patterns of certain muscles in the beef carcass also may
show an abrupt change in growth rate at birth.
Proximal hindlimb and spinal muscle groups (group 5) are nearly all
either monophasic low, monophasic average or biphasic high-average
impetus muscles.
The only group 5 muscles that reach a monophasic high level of
growth are the tensor fascia lata and the obliquus abdominis
internus. A possible explanation is that the abdominal support
provided by these two muscles might continue to expand in response
to continued visceral growth.
Distal hindlimb muscles (mostly group 3) and distal forelimb
muscles (mostly group 2) have either a monophasic low or a biphasic
low-average impetus. The requirement for locomotion immediately
after birth may be responsible for an early prenatal acceleration
of growth in these muscles, so that subsequent postnatal growth is
slower than in the remaining more proximal muscles. Since many of
the distal limb muscles have a complex pennate structure,
constraints resulting from muscle structure might also be involved.
Pennate muscles are restricted in their potential for growth,
whereas overlying muscles that are composed of relays of
intrafascicularly terminating fibers are able to grow radially and
longitudinally for a longer period.
The proximal forelimb muscles (mostly group 4 muscles) are
mostly either monophasic low or average impetus muscles. Monophasic
high and diphasic average-high impetus muscles are in the
thorax-forelimb and neck-forelimb groups. This might be a relic of
the heavy head and neck muscles that wild bulls once used for
fighting. Rams also show considerable growth of their neck muscles.
Since many of the ventral neck muscles act as antagonists to the
ligamentum nuchae, it is possible that this is an ontogenetic,
rather than a phylogenetic feature. In Zebu cattle (Bos indicus)
the shoulder hump is composed primarily of the rhomboideus cervicis
muscle. The growth of the skull and the ligamentum nuchae are
probably interrelated so that the enlargement of ventral neck
muscles is required to counterbalance a stronger ligamentum nuchae.
The distribution of the carcass muscle mass is changed when steers
lose weight on a low plane of nutrition.
Pigs
Pietrain pigs may have greater muscularity in the loin and
hindlimb, while Large Whites have relatively greater development of
neck, shoulder and forelimb muscles. These two breeds do not differ
in bone distribution. In pigs, sex and level of feeding have only
a small effect on the proportion and distribution of muscle and
bone. Differences in muscle weight distribution under hormonal
control might be caused by differences in skeletal growth.
Sheep
Breed differences in muscle weight distribution also may occur in
sheep. Ewes may have a lower percentage of shoulder, shank and neck
than wethers. However, in sheep, sex differences in muscle weight
distribution may be absent in situations where breed differences do
exist.
Cattle
In cattle, muscle distribution is influenced more by sex than by
breed. Proximal hindlimb and abdominal muscles are heavier in
heifers than in steers, and heavier in steers than in bulls. The
order is reversed for muscles of the neck and thorax. In cattle,
castration causes a marked decrease (up to 55%) in the growth of
shoulder muscles and the effect is centered on the splenius muscle
at the cervical-thoracic junction.
Many carcass muscles may acquire appreciable amounts of
intramuscular fat in older animals. This cannot be removed by
dissection and is, therefore, included in the muscle weight.
Fortunately, growth gradients for intramuscular fat in different
muscle groups are similar to those for the muscles.
When expressed on the same basis (weight of muscle plus bone),
heifers are generally fatter than steers, and steers are fatter
than bulls. These differences are related to the time of onset of
fat deposition. Since the anatomical distribution of muscle mass in
different breeds of cattle is fairly constant, the genetic
reduction of fat content probably provides the best means of
selecting for an increased proportion of lean meat.
Poultry
Allometric growth is quite conspicuous in the muscles and bones
of poultry, although not along the vertebral column as in mammals.
In ducks, the leg
""muscles are well developed at an early age, while the growth of the
breast muscles is quite late. The same overall pattern of growth
occurs in chickens and in turkeys, but to a less
extreme degree. This pattern of muscle development is an obvious
advantage to an animal that, in the wild state, would depend on its
legs for early locomotion and only later would learn to fly.
Poultry exhibit considerable variability in their body proportions
between breeds.
In chickens and turkeys, the early deposition of subcutaneous
fat is a desireable trait, but in ducks and geese the reverse is
true. Genetic selection for live weight gain is difficult to
uncouple from increased fatness. The heritabilities of the weight
and the proportion of the breast muscles are relatively high in
ducks. But, because of the marked allometry of muscle growth in
ducks, selection for gain in the breast muscles may not improve the
meat yield of the leg.
Summary
- The genetic inflexibility of the allometric growth
patterns of beef muscles discourages any attempt to change the
distribution of muscles in the carcass.
- In cattle, another
tactic is more attractive, namely, selection against fatness
throughout the carcass.
- In poultry, large allometric growth
gradients extend along the breast-leg axis, but we are not yet sure
how they will respond to genetic selection. Perhaps leg muscle
weights have a lower heritability than breast muscle weights
precisely because they are at the low end of an allometric growth
gradient.
Allometry and domestication
The domestication of farm animals several thousand years ago was
accompanied by allometric changes in body shape and by an overall
decrease in body size, but the archeological record of animal
domestication is difficult to interpret because traces of different
species may overlap.
In cattle, for example, the bones of the ancestral wild auroch (Bos
primigenius) may give way to those of the extinct shorthorn of the
Iron Age (Bos longifrons) and finally to the ancestors of our
present species (Bos taurus). The bones of B. longifrons, however,
might simply have been those of B. primigenius cows. The frequency
of occurrence of bones may reflect the balance of hunting,
containment and progressive breeding at any point in time. The
identification of individual bones is confounded by the non-random
nature of specimens left by hunters, and by technological practices
such as castration and the development of herds of cows. Selection
may have encouraged the development of paedomorphic forms with
persistent juvenile characteristics, such as pliable behavior, as
well as neotenic forms with precocious reproductive development.
Superimposed on these genetic changes there may have been changes
in body structure associated with the new environment created by
animal husbandry.
Domestication was associated with considerable reductions in the
weight of the brain; a 24% reduction in sheep, a 33% reduction in
pigs and a 16% reduction in horses. The diminution of brain size
relative to body weight was accompanied by allometric changes
between parts of the brain. In sheep, the cortical reduction of
white matter exceeded that of the gray matter, and the parts of the
brain that were involved with the senses of smell, sight and
hearing showed the greatest reduction, perhaps in relation to a
safer environment and controlled feeding. Pigs that have reverted
to a wild state do not restore their original brain size, although
allometric changes may occur: feral pigs have a smaller cerebellum
and a larger medulla oblongata.
Domestication is accompanied by allometric changes in the
endocrine glands. The relatively recent derivation of laboratory
rats from wild Norway rats (Mus norvegicus) provides an interesting
model of events that may have occurred during the domestication of
farm animals. The dominant changes in rats were:
- a reduction in the adrenal cortex,
- and an increase in the activity of the gonads.
In wild and domesticated ducks, appropriate differences occur in
the pituitary-adrenal system, but the nature of the relationship
between domestication and changes in behavior and adrenocortical
activity is still open to question.
Sheep provide a striking example of phenotypic changes that may
be produced by what Charles Darwin called unconscious selection.
These changes include:
- (1) the ratio of wool to hair,
- (2) an increase in tail length or adiposity,
- (3) development of the lop-eared condition,
- (4) increased convexity of the nose due to a decrease in jaw
length, and
- (5) a reduction in the number and complexity of horns.
Another factor that may be involved in the interaction of animal
breeding with growth is the general trends towards an inverse
relationship between functional maturity and growth rate in muscle.
Thus, muscles that must be able to function effectively for
locomotion at birth may not be able to grow rapidly, and vice
versa. This has been demonstrated in different species of birds
and could also be operative in domestic animals. In splayleg
piglets, for example, perhaps selection for rapid muscle growth has
reduced functional maturity of the muscles at birth.