9 Neurotrophism
9.1 Introduction
We have seen how myoblasts fuse to form multinucleate myofibres
containing contractile myofibrils. A key point was the first myoblasts
fused to form myotubes in the centres of their fasciculi (bundles of
myofibres) while later myoblasts fused to form secondary fibres towards
the outside of their fasciculi. In the pig, we saw how the myofibres
located centrally in their fasciculi were likely to be slow-contracting
myofibres with strong aerobic activity, while myofibres located on the
outside were likely to be fast-contracting with strong anaerobic
activity.
Thus, in the pig, myotubes are
likely to develop into myofibres with slow-contraction and strong
aerobic activity while secondary fibres develop fast-contraction and
strong anaerobic activity. This is generally true, but subject to
modifications considered in this lecture.
Neurotrophism is one of the
names used for the control of myofibre metabolism (both contraction
speed and the balance of aerobic and anaerobic metabolism). Thus,
neurotrophism is important in explaining muscle development. But first
some questions.
Why is muscle fibre metabolism
important for understanding meat production? Because fast-contracting
myofibres exhibit fast growth, which means rapid meat production and
high meat yields. And because slow-contracting myofibres are
associated with taste and juiciness - which are obviously important in
understanding meat quality.
Why do we use the pig as a model? Because in the other species of
meat animals the different types of myofibres do not maintain their
positions in the fasciculi - their arrangement gets randomized and it
is difficult to see what types of myofibres are derived from myotubes
and secondary fibres. The reason why the pig is so neat and tidy
in this respect is unknown. Historically, it gave animal
scientists an advantage over biomedical scientists working with other
animals - and we were able to make some useful contributions in this
field using the pig as our model. Perhaps it was no accident the great
German histologist, Theodore Schwann, used pigs as his material for
proving animal bodies are composed of cells (the Cell Theory is now so
obvious and widely accepted we forget what a major discovery it was).
This is why he had to investigate the origin of skeletal muscle -
because myofibres were the only cells of the body with many
nuclei. His theory was the body is divided into cells - each with
one nucleus. Thus, he had to explain the special case of multinucleate
myofibres - and he did so brilliantly, showing this was a special case
where cells had fused together.
9.2 Trophic effects
- Motor
neurons exert a long term regulation over the physiological and
metabolic
properties of all the myofibres in a motor unit. This is often called
the trophic
effect of nerve on muscle. The word trophic implies something of a
nutritive
effect, as if the nerve was feeding the muscle, but its current usage
sometimes
includes possible non‑nutritive effects such as the frequency patterns
of nerve
impulses to the muscle. The idea nerves might have a trophic
function is
far from new, and probably originates from ancient observations on the
degenerative fate of denervated organs.
- All myofibres in the same motor unit have the same
histochemical properties - because they are all
innervated by the same motor neuron. This is true for our meat
animals, but not for other species such as lobsters.
- Trophic effects may be bi‑directional and sometimes the
muscle has an influence on its motor neuron. For example, connections
on the perikaryon (presynaptic terminal boutons)
are lost when axons are cut, and they are restored when neuromuscular
contact
is re‑established. Similarly, growth-regulating substances may pass
from muscle to nerve.
- Denervation by cutting the axon linking the motor neuron
to its myofibres removes the trophic effect - and the myofibres wither
away (atrophy).
9.3 Crossed-reinnervation experiments
When axons are cut
they can grow back to their motor unit - probably by the same
mechanisms they first used to grow out to, and innervate the myofibres.
Thus, a denervated motor unit can be restored to almost normal function
if its axon is able to reconnect. In a reinnervation experiment two
muscles are selected in the limb of an animal - one is dominated by
slow-contracting myofibres and the other by fast-contracting myofibres.
The nerves to these two muscles are cut and allowed to grow back.
We check normal function returns. The slow muscle is still slow,
and
the fast muscle is still fast. Gaining confidence with the
technique we then attempt a crossed-reinnervation
experiment. The two nerves are cut and crossed over. The slow nerve is
connected to the fast muscle. The fast nerve is connected to the
slow muscle. What happens? When the axons grow back, they cannot
find their original myofibres. Instead, they reinnervate the myofibres
they can find. The slow muscle
then becomes fast-contracting, and the fast muscle become
slow-contracting. The transformation is often imperfect but
enough for all to agree there has been a change in contraction speed.
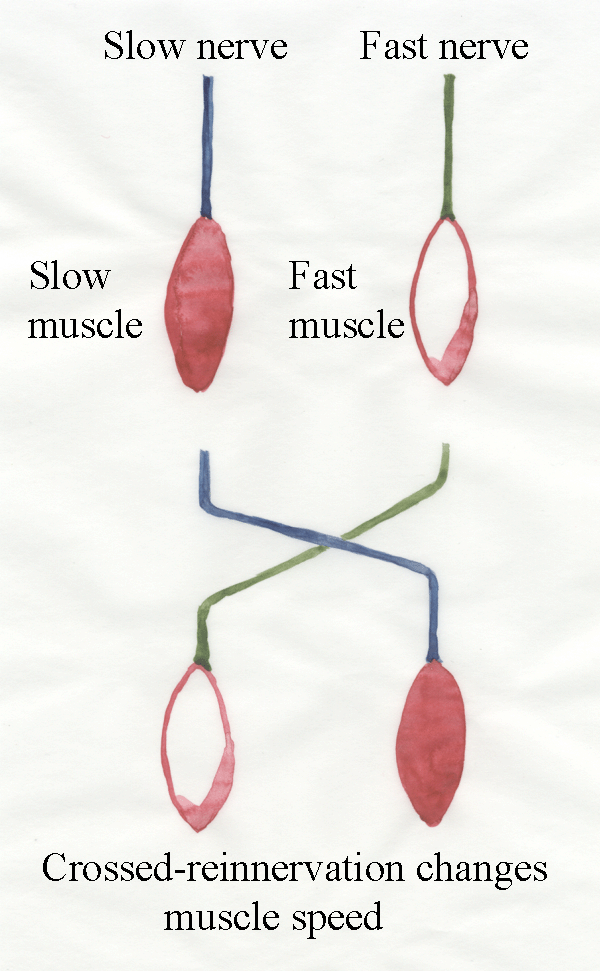
- The nerves normally
innervating fast muscles may differ from those normally innervating
slow
muscles in two general ways: (1) in the physical or chemical nature of
the trophic
factor they might deliver, and (2) in the frequency profile of the
impulses they normally convey. These two possibilities are as difficult
to separate as the effects of denervation and disuse. There is
independent
evidence for both, but they might also be interdependent.
For example, impulse frequency might
influence the rate of delivery of trophic substances .
- Similar results
to those of crossed reinnervation experiments may be obtained by the
transplantation of muscles. A fast muscle regenerates in the
original
location of a slow muscle and vice versa. This confirms the validity of
crossed-reinnervation experiments.
9.4 Axoplasmic flow
Trophic factors appear to be
released from nerve terminals, often at a great distance from the
perikaryon.
But where are trophic substances produced in the neuron? Motor axons
grow
towards their muscles when neuromuscular relationships are first
established
and this involves a great increase in axoplasmic volume. The source of
new
axoplasm in the perikaryon is sufficient to allow repeated regeneration
of
transected axons, no matter how often they are cut back. If axons are
constricted by a tight external ring or "bottleneck", the movement of
axoplasm down the axon causes a bulge to develop proximal to the
constriction.
Distally, the axon becomes narrower because of a reduction of the
axoplasmic
flow. Thus, there is an axoplasmic flow along axons away from the
perikaryon and axoplasmic flow is somehow involved with neurotrophic
effects.
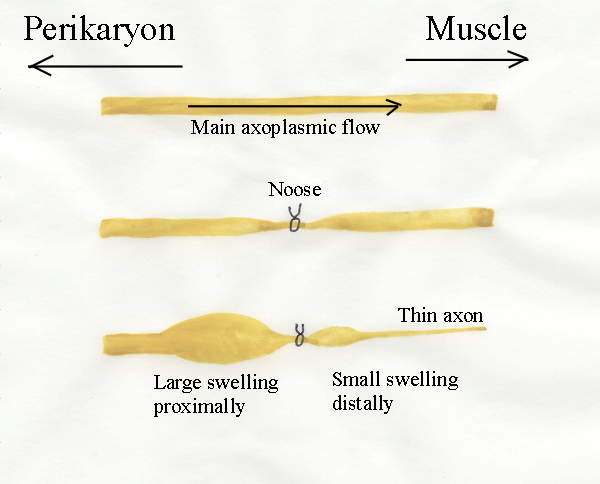
- Axoplasmic flow in mature
axons is a bi-directional process with
both slow (1 mm/day) and fast (400 mm/day) streams.
- Slow
transport probably accounts for the growth and regeneration of axons
since the
amount of material conveyed by slow transport greatly exceeds that
carried by
fast transport.
- Fast transport is channeled in some
way by microtubules or vesicles in the axon, and is sensitive to oxygen
and
temperature levels. Colchicine disrupts microtubules and
interferes with axoplasmic flow. It causes muscles to exhibit some of the features of denervation.
- Slow transport carries mostly high
molecular weight soluble proteins, while fast transport carries
particles and a
small amount of soluble protein.
9.5 Activity patterns
One of the great difficulties of investigating
neurotrophism is fast nerves exhibit burst of intense activity while
slow nerves exhibit continuous low levels of activity. Think of
your own body movements, You use your fast muscles to do something
vigorous now and again, while you use your slow muscles
continuously for breathing and posture. Experimentally changing
these activity patterns by blocking all normal activity and
superimposing artificial activity from a programmed stimulator may
also change myofibre metabolism.
9.6 Changes During Growth
Histochemical types are important in meat animals because they are
related to muscle growth and
meat quality. Histochemical types also react differently during the
conversion of muscles to meat, because they contain different levels of
glycogen and anaerobic enzymes. Before it became known that myofibres
could
change from one type to another, growth-related changes in fibre types
were not adequately controlled in agricultural experiments using
myofibre
histochemistry. Be very
critical of any research paper assuming fibre
types are constant regardless of animal age!
This three dimensional plot shows changes in myofibre
histochemistry during muscle growth in a pork muscle. In the bottom
right corner, a population of fast-contracting myofibres (alkaline
ATPase +) which are also anaerobic (anaerobic +) is changing to develop
more aerobic activity (from 0 to + aerobic). Then their speed of
contraction decreases (from 0 to + for acid-stable ATPase and from + to
0 for alkaline ATPase). This type of change happens in
older pigs as they become fatter. The initially white muscles along the
loin develop more pigmentation as progressively more time is spent in
postural work. Thus, meat from old pigs is more pigmented than
meat from younger pigs. This is true for many meat animals. Thus,
beef is more pigmented than veal, and mutton is more pigmented than
lamb.
Here are some important examples of
growth-related changes in meat production.
- Splayleg piglets.
Piglets are very vulnerable just after farrowing. The sow is likely to
sit on them and crush them if they cannot move out of her way. Movement
requires fully functional limbs. If the hindlimbs splay outwards,
locomotion is impossible. What normally holds the hindlimbs together?
Answer - the medial adductor muscles.
Immediately after farrowing there should be a rapid recruitment of
postural myofibres. Intermediate myofibres are converted to red
myofibres. This does not happen in splayleg piglets. The
hindlimbs should be loosely tied together with twine. This enables the
piglets to scrabble along and survive until adductor muscle function
becomes adequate.
- Rapid muscle growth in young
animals. Rapid muscle growth (highly desired by
producers) occurs because white myofibres exhibit faster increase in
size (myofibre hypertrophy)
than intermediate or red myofibres. ALSO, intermediate myofibres are
converted to white myofibres.
- Slow muscle growth in fat
animals. Slow muscle growth in the finishing period
(highly desired by consumers who like tasty, succulent meat) occurs
because of the increasing postural demands placed on major locomotory
muscles. The animal has become fat and heavy. It no longer leaps
around like a baby. It is slow moving. Intermediate myofibres are
converted to red myofibres. This is why meat from older animals
is dark in colour (higher myoglobin) and strong in taste (more lipid).
- White meat in poultry.
Chickens and turkeys evolved from heavy, grain-eating birds. They
only used flight to escape from predators. For this they require
massive, powerful wing muscles. Thus, chickens and turkeys are
genetically programmed to develop breast muscles dominated by white
myofibres derived from both secondary fibres AND myotubes. This is the only case where we
find myotubes becoming white myofibres.
Further information.
Structure and Development of Meat
Animals and
Poultry. Pages 324-336. Trophic effect of nerves.
Trivia
The first crossed re-innervation experiments were undertaken in late
Victorian times - but the researchers failed to notice the change in
contraction speed. The subject started up again in the 1940s to help
with nerve regeneration in young soldiers.The transformation of
histochemical types of myofibres is declining in research popularity -
which is a good thing in my opinion. Most researchers relied too
heaving on subjective typing of myofibres (it's not real science unless
you can measure it quantitatively!) and totally ignored the way
intrafascicularly terminating myofibres can bias the data. The last
blue diagram is where I quit the field 20 years ago.