13 Rigor & Cold shortening
13.1 Introduction
Rigor mortis is a loss
of muscle extensibility marking the conversion of
muscle to meat. In other words, living muscles can be stretched and
they return to their resting length when released. Meat cannot be
stretched and has very little elasticity. A strong attempt to
stretch a length of meat will merely rip it.
Just before a muscle sets in rigor mortis, it may attempt to shorten.
Refrigeration increases the shortening - giving rise to the name cold shortening. But even
cold shortening is weak relative to contraction of a living
muscle. A muscle will only contract if there are no skeletal
restraints. What does this mean? Consider a beef animal
walking into the abattoir on all four legs - a leg at each corner of
the body. We slaughter it and suspend it by its hindlimbs.
The muscles ventral to the vertebral
column are stretched and cannot
shorten before rigor mortis develops. But the muscles dorsal to the vertebral column
have no skeletal restraints and are
free to contract - either from the very weak shortening
just before rigor develops, or the slightly stronger cold shortening
caused by refrigeration.
Why is this important? Because shortening decreases sarcomere length and increases the overlap of thick and thin
myofilaments. This increases
the toughness of the meat. Nobody likes tough meat.
It is important to understand how to minimize cold-shortening.
The key point to grasp is - the
sarcomere can only shorten if it still
has ATP and has not yet developed rigor mortis. An exhausted muscle has
minimal glycogen, therefore minimal post-mortem re-synthesis of ATP,
therefore it develops rigor mortis early. Once rigor has developed -
the muscle cannot shorten. We are safe. We cannot make meat
tough by rapid refrigeration.
Cold-shortening is a very complex phenomenon. The most likely
cause is the effect of low temperature on the sarcoplasmic reticulum.
The sarcoplasmic reticulum works hard using ATP to sequester calcium
ions. When it is cooled - it begins to fail. Calcium ions remain
in the cytosol. The muscle slowly contracts. Cold
shortening is more severe in red muscles than in white muscles because
red muscles have a weak sarcoplasmic reticulum.
13.2 Rigor mortis
The conversion of muscles to
meat is completed when muscles have depleted their energy reserves or
have lost
the ability to utilize remaining reserves. In living muscles at rest,
an ATP
molecule binds to each myosin molecule head and in this condition the
myosin
head is said to be "charged". In resting muscle, further developments
between the actin and myosin of thin and thick myofilaments are
prevented by the
intrusion of tropomyosin
molecules. Contraction in living muscle
is initiated by the release of calcium
ions from the sarcoplasmic
reticulum,
and followed by the removal of the tropomyosin intrusion. As
a muscle contracts, charged
myosin molecules heads attach to actin molecules, ATP is split to ADP with a
release of energy, and the myosin molecule head
swivels to cause filament sliding. The myosin molecule
head, which is still
attached to its site on the actin, can only detach itself if a new ATP
molecule
is available to be be bound. When muscle is converted to meat, myosin
molecule
heads remain locked to actin and even passive filament sliding is
impossible.
13.3 Rigorometer
A rigorometer is a device for
measuring the loss of extensibility as rigor mortis develops. The first
rigorometer was developed in the late 1930s by one of the pioneer meat
scientists (Bate-Smith) and was used extensively by another pioneer
(Bendall). The Rigorometry gently loads the bottom of a hanging muscle
strip and
records
the resulting extension. If a suitable load is placed on
a muscle
strip still containing ATP, the free end drops as the muscle stretches
and,
when the load is removed, the muscle returns to its original length.
But when
ATP is no longer available, the muscle is only very slightly
extensible. Muscle
strips are maintained in an anaerobic atmosphere to prevent
aerobic surface
resynthesis of ATP.
For a short time after
exsanguination, ATP may be resynthesised from CP. After CP has been
used up, the
length of time before the occurrence of rigor mortis depends on the
amount of
glycogen available within the muscle and on the survival of glycolytic
enzymes.
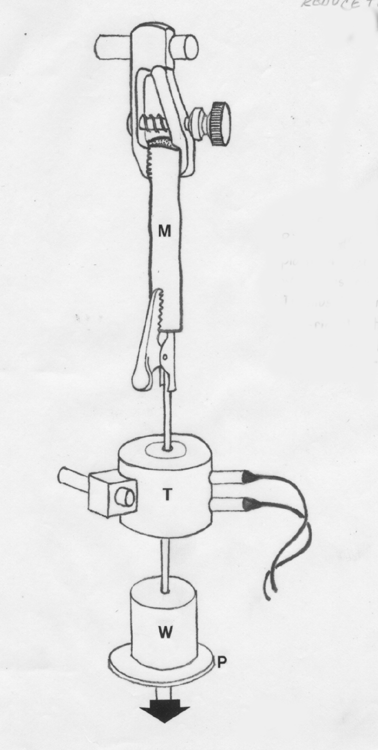
In
the diagram above, the muscle strip (M) is loaded with a weight (W) as
the platform (P) drops. Whether or not the muscle extends when loaded
is detected by the transducer (T).
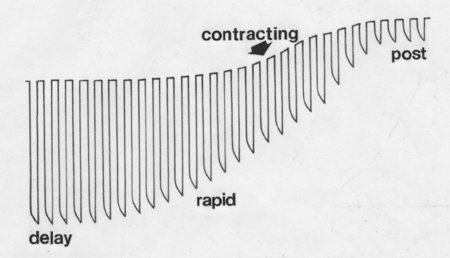
A number of
features appear
on a rigorometer trace (as above). The delay
period is when ATP is
still
being resynthesised, the rapid
phase is when individual myofibres
run out
of ATP, and the final post-rigor
phase is when a sufficient number of myofibres
have set in rigor mortis to prevent any further extensibility.
Sometimes, the
muscle may slowly and weakly contract as it goes into rigor. The
behavior of
muscle strips in a rigorometer and of whole muscles in a carcass, is
greatly
affected by the condition of the animal at the time of slaughter. These
differences may be seen on the rigorometer as follows.
(1) An animal calm
during slaughter gives a long
delay, a slow rapid phase,
and a decrease
in
unloaded length at body temperature but not at room temperature.
(2) An animal struggling
during slaughter gives a short
delay, a short rapid phase,
and a
decrease in
unloaded length at body temperature but not at room temperature.
(3) An exhausted
animal gives an
extremely short or non‑existent delay
period, a very short rapid phase,
and
shortens its unloaded length at both
body and room temperature.
(4) A starved animal
gives a
short delay period, a fairly long rapid phase, and a decrease in
unloaded
length at body temperature but not room temperature.
-
Certain muscles may attempt to shorten as they set in
rigor mortis,
depending on animal condition at the time of slaughter and on muscle
temperature (superficial muscles will
cool faster if not extensively
insulated
by fat).
-
Some of these muscles will be completely prevented from
shortening
because they are already stretched by the weight of the hanging carcass
but unrestrained muscles, such
as the posterior hindlimb muscles of a
hanging
carcass, are free to contract.
-
If rigor contraction is stronger than
normal, the position of the forelimb
may
be raised against gravity.
-
Unrestrained carcass
muscles contracting
as they
develop rigor produce meat with a shortened sarcomere length, a greater
overlap
of thick and thin filaments, and a greater number of rigor bonds
linking the
filaments.
-
Meat in this condition is less tender and often has a decreased
water binding capacity.
- The extent of rigor
contraction of unrestrained
muscles
may be reduced by hanging carcasses from the pelvis. However, retail
cuts of
meat may lose their familiar appearance, the normal tenderization by
stretching
may be lost in certain muscles, and carcasses may be difficult to
handle on a
normal overhead rail system.
- The major
problem with
a laboratory rigorometer is removing a muscle strip from the
carcass
changes its properties, mainly because of increased excitation.
However, subsequent research using other types of rigorometers on whole
carcasses shows a similar pattern of results.
13.4 Electrical stimulation (ES)
- Electrical stimulation (ES)
of carcass muscles soon after slaughter accelerates
their normal decline in pH and may enhance tenderization during
conditioning.
- Although there was some early work on ES in the US,
commercial possibilities
did not become apparent until it was shown in New Zealand that
stimulation
prevented COLD Shortening in lamb.
- ES now is widely used for BEEF and LAMB. There are
some who
consider it can be used for pork, although care must be taken to
prevent
PSE.
- As well as protecting against cold shortening, electrical
stimulation may
improve
- meat tenderness,
- color and appearance, and
- subjective scores for youthfulness used in meat grading.
However, cooking losses may be increased by ES. - Apart
from guarding against cold shortening, the beneficial
effects of ES
on meat tenderness could also involve myofibre fracture. In
other words, when the muscle contracts violently after slaughter it may
rip up the myofibres making them more tender.
- ES is ineffective on dark-cutting beef (caused by
beef having little or no glycogen and minimal post-mortem glycolysis)
and ES is of no value without an accelerated decline in pH. In other
words, dark-cutters have little or no glycogen after slaughter, so they
cannot make much lactic acid, so they cannot benefit from advanced
glycolysis
- ES may turn on some of
the enzymes that make meat more tender during the conditioning period.
- Different voltages have been used: from 32 to 1600 V.
- Different amperages have been used: from 0.5 to 6 A.
- Types of electrode have included
- aluminum foil
- probe or pin types
- rectal probes
- multipoint electrodes
- hooks and shackles
- Electrode positions usually have spanned great lengths of
carcass
from neck to hindlimb, often with electrodes in muscles but sometimes
in
the spinal cord. Contralateral differences in the effectiveness of ES
may be detected when one hindlimb is shackled and the other side moves
freely.
- The time delay between exsanguination and stimulation has
been extended
up to 60 minutes, during which time carcasses have received variable
treatments
such as evisceration and splitting. Stimulation during exsanguination
is not effective.
- Impulse frequency and duration of application range from 3 to 400
Hz. Muscles
differ in the optimum frequency required for their maximum stimulation.
- Wave forms of stimulatory impulses have included
- square waves of interrupted direct current
- unchanged or partly modified sinusoidal waves of alternating
current.
In summary: higher voltages are
required the longer the delay between exsanguination
and ES.
13.5 Involvement of the nervous system.
Even in a simplified laboratory model of
carcass stimulation, with a muscle strip and a pair of stimulatory
electrodes,
the response may be quite complex. Although stimulation
may accelerate post mortem metabolism, muscle with an already
accelerated
rate of metabolism may lose its excitability at a faster than normal
rate.
Thus, animals with intrinsically fast glycolytic rates may be
detected
by their reduced electrical excitability.
Unless special precautions are taken to the contrary, muscle strips
contain severed intramuscular nerves and neuromuscular
junctions among the myofibres. Immediately post mortem, all three
components may be excitable with their own particular activation
thresholds
and, as these change post mortem, it is difficult to identify the point
between the axon and the myofibre responding first to ES.
The complete final common pathway from the spinal
cord to the muscles survives for many minutes post mortem.
The excitability of muscle strips decreases progressively post
mortem
so that either a higher voltage and/or a longer duration stimulus is
needed
to obtain a constant response, and it is likely that the initial loss
of
excitability is caused by fatigue in the excitation-contraction pathway.
If neuromuscular junctions are pharmacologically blocked in samples
taken
shortly after animal exsanguination, excitability is decreased. This
suggests the high excitability of muscle strips at this time is
caused by intramuscular
motor axons and/or their neuromuscular junctions.
ES and Rate of Stimulation
Living muscles or strips taken immediately after animal exsanguination
respond to a progressive increase in stimulus frequency by twitching at
a correspondingly faster rate, until the twitches merge into a
sustained
contraction or tetanus, as shown below.
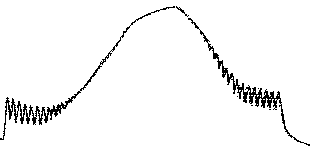
As the time between animal exsanguination and muscle stimulation is
increased, muscle strips become progressively less able to maintain
tetanus, as shown below.
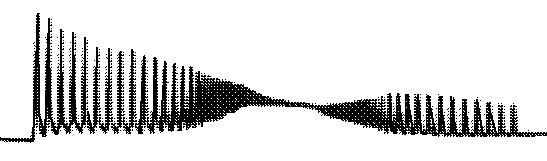
Immediately after the excitation of axons and myofibres, there
follows
an absolute refractory period of complete inexcitability to a
second
stimulus since the response to the first stimulus is still in progress.
Next comes a relative refractory period when, if the second
stimulus
is of sufficient magnitude, excitation may be elicited. The duration of
the relative refractory period probably increases progressively post
mortem.
Many of the stimuli delivered at a high frequency may, therefore,
arrive
during a relative refractory period and elicit no response. For this reason,
the stimulus frequency for ES of carcasses is usually kept low.
As shown in this example,
impulses arriving at 5 impulses
per second produced a stronger effect (line goes up, indicating muscle
contracted) than impulses arriving at 10 impulses per second.
The pH decline in meat post mortem may render meat less excitable to
ES
via the nervous system since a low pH reduces the amount of
acetylcholine
released at the neuromuscular junction. The accumulation of calcium
ions
by transverse tubules might also be involved in the decreased
excitability
of muscles after ES.
Red & White Muscle
White muscles have a greater response to ES than red muscles,
because
anaerobic
myofibres respond more readily.
The higher rate of glycogenolysis in white muscles is caused
by a high content of phosphorylase, greater activation of phosphorylase
and a higher content of creatine phosphate.
The effects of ES on meat are not limited to the actual
period
of stimulation, but persist afterwards, perhaps because of
changes
in the sarcoplasmic reticulum.
Electrical stimulation causes swelling of
the sarcoplasmic reticulum, transverse tubules and mitochondria,
together
with autolytic ultrastructural changes. Increased binding of glycolytic
enzymes to actin filaments also may be involved.
Red and white muscles also differ in the way in which temperature
affects the activity of the sarcoplasmic reticulum.
ES and Current Pathway
Current pathway is difficult to assess in whole carcasses. In
homogeneous
conductors, resistance is proportional to the distance between
electrodes,
and is inversely proportional to conductor cross sectional area.
In homogenous conductors, resistivity (resistance specific to conductor
material) normally is measured between opposite faces of a one
centimeter
cube. However, not only are carcasses interrupted by tracts of fat
with
a high resistance and by bones with a variable resistance, but
muscles
themselves are electrically anisotropic (meaning the direction
across which measurements are made affects the results). Resistivity is inversely
proportional to meat temperature, it tends to be greater across rather
than along myofibres, and it may show a transient increase post mortem
followed by a progressive decline.
Resistance of a whole carcass is modified by factors such as,
- the time lapse between animal exsanguination and muscle
stimulation,
- the distance between electrodes,
- electrode surface area in contact with meat or connective tissue
and not
blocked by fat,
- whether or not the carcass is whole, eviscerated or split, and
- electrode location.
Continuous DC currents only elicit strong stimulation when they are
first
applied, but interrupted DC currents of square wave impulses may be
used
to prolong this initial response. However, DC currents of any type soon
cause Polarization at the electrode-tissue interface. Polarization
increases
resistance and decreases responses. A muscle that has almost ceased
responding
to unidirectional square waves may respond with original intensity if
polarity
is reversed, as shown below.
.
To avoid polarization, square
waves of alternating polarity may be used but apparatus to produce
these
at high voltage may be expensive. Thus, there are some advantages to
using
apparatus which supplies modified sine wave impulses derived from the
regular
50 or 60 Hz commercial power supply.
13.6 Summary
- Rapid refrigeration of meat after slaughter reduces the risk
of dangerous bacteria on the meat and is generally a good thing.
- But rapid refrigeration may cause sarcomeres to shorten, thus
making the meat tough.
- Electrical stimulation is used to deplete ATP, thus initiating
the early development of rigor mortis.
- Once meat is set in rigor mortis it cannot shorten any more and
low temperatures have no effect on tenderness.
- If meat is NOT electrically stimulated, the usual generalization
is it will cold shorten if it gets below 10º C with 10 hours of
slaughter.
Further information
Structure and Development of Meat
Animals and Poultry. Pages 553- 563.