12 Glycolysis
12.1 Introduction
Glycolysis is the
decomposition of glucose by
hydrolysis. Most of the glucose in muscle is stored as glycogen. Thus , glycolysis is also
the decomposition of glycogen. Glycolysis is supremely important
in understanding how muscles are converted to meat. Glycolysis normally
causes an increase in acidity (= decrease in pH) as muscles are
converted to meat. Too much or too little acid - and the meat is
ruined.
IF you have not taken a course in biochemistry - concentrate on
section 12.2 to 12.5. If you are bored by yet another repetition
of glycolysis have a look at sections 12.6 onwards.
12.2 Living muscle
- In living muscle, the complete
oxidation of glucose to carbon dioxide
and water requires oxygen, and it releases a lot of energy. Much of
this
energy is captured by adding a phosphate group to another molecule that
already has two phosphate groups. Thus, adenosine diphosphate (ADP) is
converted to adenosine triphosphate (ATP).
- The ATP molecule carries this
energy within the myofibre, and it may be released to another
biochemical
system by cleaving off the added phosphate.
- Muscle contraction
is a primary user of ATP in the living animal, but substantial amounts
of ATP are used by the membranes around and within the myofibre for
maintaining
ionic concentration gradients.
- One molecule of glucose may be combined
with oxygen to add a phosphate group to each of 36 molecules of ADP.
- In
addition to 36 molecules of ATP, this produces carbon dioxide and
water.
- However, if the muscle is
without oxygen (anoxic) after
slaughter, the
most it can normally gain by oxidizing each glucose unit is two
molecules of ATP.
12.3 Glycogen
Structure of a glucose unit with its carbon atoms numbered is shown
below.
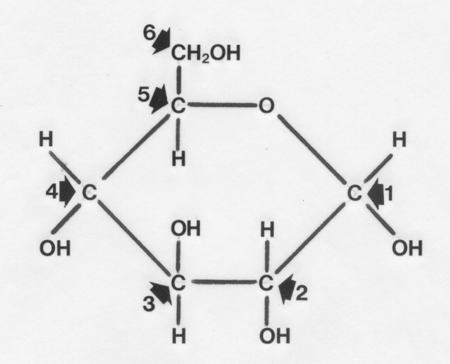
These are joined by straight 1-4 linkages or branched 1-6 linkages to
form glycogen.
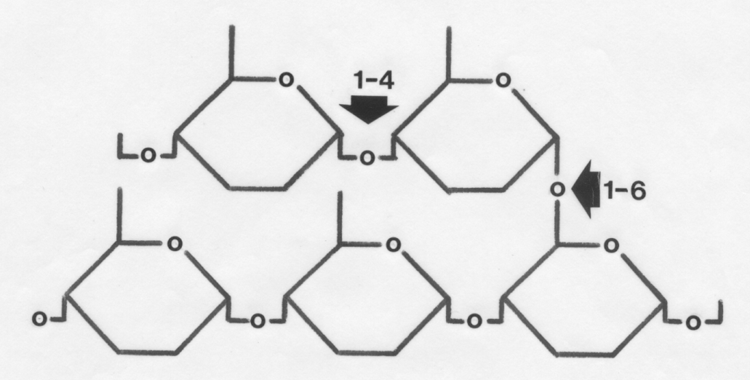
- Glycogen is the primary storage carbohydrate
in myofibres and appears in electron micrographs as single granules
or clumps of granules located in the sarcoplasm (cytoplasm) between
myofibrils and under the cell membrane.
- Scanning
tunneling microscopy (one of the most powerful types of microscope yet
invented) reveals glycogen granules are ellipsoidal with a laminar
structure which suggests they grow from one edge rather than a central
point.
- In longitudinal sections of skeletal muscle, glycogen and its
associated
enzymes are concentrated at the I
bands (one of the transverse bands across
the muscle fibre when examined microscopically).
- Glycogen is a polysaccharide
formed by the linking together of large
numbers of glucose units. However, the glycogen from some animal
tissues
is a proteoglucan
(glycoprotein) that may contain other monosaccharides
and phosphate ester groups.
- The concentration of glycogen in foetal muscle increases steadily
towards the end of gestation and peaks at the time of birth. Pigs have
very high muscle glycogen levels at birth but they decline rapidly
after
birth to reach future adult levels within a week.
- Glycogenin is a protein
acting as a starting point for the formation of new glycogen.
The image below shows a transverse section of pork stained by
the periodic acid-Schiff reaction for glycogen. All the myofibres
started with glycogen, but most myofibres have depleted their glycogen
during the conversion of muscle to meat. Note how some of the myofibres
have a central core of glycogen.
12.4 After exsanguination
- Once the animal has been exsanguinated,
the oxygen within the muscles is
rapidly exhausted, and the process of converting muscle to meat really
begins.
- Glycogenolysis is the
enzymatic degradation of glycogen,
and is the first step in the release of energy by the oxidation of
glucose
units.
- Phosphorylase erodes
straight chains (from their non-reducing ends at carbon 4) and attaches
a phosphate group to the carbon atom at position 1 as it removes a
glucose
unit.
- Phosphorylase erodes straight chains until it comes to the fourth
glucose unit preceding a branch point.
- The three glucose units before the
fourth one carrying the branch are removed together, and are added
to an adjacent free straight chain so the 1-6 linkage thus exposed
at the branch point may be severed.
- A second enzyme, debranching
enzyme,
performs this task.
- Instead of being released as glucose-1-phosphate,
the
glucose unit released from a branch point remains as free glucose.
- Thus, glycogenolysis
liberates glucose-1-phosphate and glucose in a ratio determined by the
mean length of straight chains and the
number of branch points.
While the structure of glycogen and the mechanism of
glycogenolysis
are important in understanding the conversion of muscles to meat, the
remainder
of the pathway by which glycogen is anaerobically oxidized to lactate need
only be covered in general principle. The most important question to be
answered is why lactate is produced under anaerobic conditions, yet
hardly
at all under aerobic conditions.
- After a series of steps in the glycolytic
pathway, molecules with six carbon atoms derived from the glucose units
of glycogen are split to produce two molecules of pyruvate, each with three
carbon atoms.
- All the glycolytic enzymes (except for hexokinase) are
concentrated
in the I band.
- If aerobic conditions prevail, pyruvate formed in the cytosol
of the myofibre now enters a mitochondrion
(the metabolic furnace of
the cell).
- After entering a mitochondrion, pyruvate is converted to acetyl-CoA
which becomes fused to oxaloacetate
to form citrate.
- The citrate then is
oxidized in the Krebs' cycle,
which is completed by the regeneration
of oxaloacetate.
- Continuous activity of the Krebs' cycle is fueled by
a range of carbohydrates, fatty acids and amino acids, and is the
primary
system for the aerobic generation of energy.
- Large numbers of molecules
of ATP are produced from ADP by a series of reactions, oxidative phosphorylation, in
the mitochondrial membrane.
- Under aerobic conditions, the production of two pyruvate
molecules from
a glucose-1-phosphate molecule results in the reduction of 2NAD+
.
- Thus, somewhere else in the myofibre, NADH must be re-oxidized for
glycolysis to continue.
- Aerobically, this occurs as a consequence of mitochondrial
Krebs' cycle activity, although NADH and NAD+ do not actually cross the
mitochondrial membrane.
- In
anaerobic living muscles and in meat, the Krebs'
cycle is halted, and NADH is re-oxidized in the cytosol by
lactate dehydrogenase
(LDH) during the conversion of
pyruvate to lactate.
- Pyruvate is of no immediate
use anaerobically since mitochondrial oxidation has ceased, but its
conversion
to lactate ensures a continued supply of NAD+ for the continuation of
glycogenolysis
and anaerobic glycolysis in the cytosol.
- However, since these events form
only the initial stages of complete carbohydrate oxidation, they do not
regenerate much ATP.
LDH adds hydrogen to
pyruvate to produce lactic acid and exists in a
number of isoenzymes separable electrophoretically by their net
electrical
charge. If LDH-1 is prevalent,
it facilitates aerobic metabolism, where
possible, since it is inhibited by pyruvate and lactate. LDH-5 is not inhibited
by high levels of lactate and pyruvate, and it facilitates anaerobic
metabolism.
LDH-1 is typical of cardiac muscle
while LDH-5 is typical of skeletal muscles,
particularly those adapted for anaerobic conditions during contraction.
In skeletal muscles, the ratio of LDH-1 to LDH-5 corresponds to the
dominant
activity pattern of a muscle. For example, muscles capable of sustained
activity and which only use aerobic metabolism have high LDH-1. LDH-5
is
the dominant isoenzyme in skeletal muscles from slaughter-weight pigs.
12.5 Regulation of glycogenolysis
- The regulation of glycolysis in a myofibre of a live animal is
integrated
with the metabolic state of the myofibre and its immediate energy
needs.
- The
metabolic state of the myofibre is profoundly affected by hormones,
particularly adrenaline, and
by the
extent of recent contractile activity of the myofibre.
- Phosphorylase is
particularly important in the conversion of muscle to
meat since it may be a primary control site for post mortem glycolysis.
- Phosphorylase in muscle is most active when it is, itself,
phosphorylated
(a-form). When dephosphorylated, it is less active (b-form).
- In general terms, therefore,
phosphorylase is switched on and off by the addition or removal of its
phosphate, with on and off states being relative rather than absolute.
- The activity of phosphorylase b
is dependent on the presence of AMP but
the activity of phosphorylase a
is not.
There are two conflicting requirements complicating the activation of
phosphorylase.
Firstly, since phosphorylase initiates the release of considerable
amounts
of chemical energy, there must be safeguards to prevent its
uncontrolled
activity. In stress-susceptible pigs,
for example, the uncontrolled activity
of anaerobic glycolysis may lead to excessive
heat production and to a
level of acidity that may soon prove fatal. The conflicting requirement
is vast amounts of phosphorylase spread through the muscle mass
must be rapidly activated by relatively small amounts of adrenaline.
The
adrenaline activation of severely frightened animals often is called
the
"fight or flight" response:
neither of these responses is likely to be
of much survival value if the anaerobic energy supply to body muscles
is
delayed.
The conflicting demands for fail-safe but rapid activation are
satisfied
by two particular features of the activation system.
- The conversion
of phosphorylase b to phosphorylase a is inhibited locally in each myofibre by high concentrations of ATP and
glucose-6-phosphate (an intermediate
in the conversion of glycogen to lactate). Thus, if the energy released
by phosphorylase is not rapidly consumed, the energy release system
shuts
down. If the energy is used, however, AMP and phosphate further enhance
the activation of phosphorylase.
- To enable the rapid activation of phosphorylase throughout the
musculature, the relatively small amounts of adrenaline arriving at
the muscle initiates a series of biochemical changes functioning as an
amplifier.
A small input leads to a large output.
- Adrenaline causes adenyl cyclase
to increase its formation, from ATP, of cyclic AMP.
- Then cyclic AMP activates protein
kinase.
- With ATP and magnesium ions present, protein kinase then
phosphorylates another enzyme, phosphorylase
b kinase b.
- The active form,
phosphorylase b kinase a, in the presence of magnesium ions, finally
activates
phosphorylase b to phosphorylase
a.
- As a final safety factor, if the supply
of inorganic phosphate is inadequate, even phosphorylase a will be
relatively
inactive but the system will be primed for rapid energy production once
muscle contraction is initiated.
Remember the sarcoplasmic reticulum?
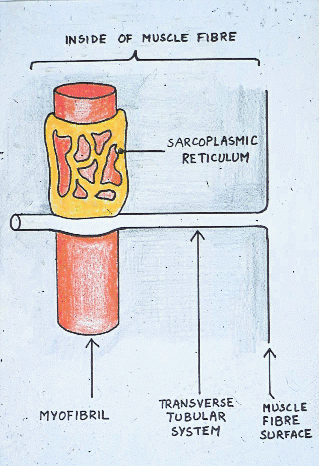
When animals require energy anaerobically during normal
activity,
phosphorylase b kinase is activated by calcium ions released from the
sarcoplasmic
reticulum - normally the trigger for muscle contraction in
living muscle.
Glycogen granules are closely related to the sarcoplasmic reticulum and
to glycogenolytic enzymes as part of a structural complex. The
activation
system linking muscle contraction to glycogenolysis is short lived to
avoid
the continuous use and depletion of glycogen reserves. Glycogen is a
rapidly
available energy source for both brief muscle activity and the early
stages
of sustained activity. Phosphorylase activity is curtailed by
phosphatase,
which dephosphorylates phosphorylase a and phosphorylase b kinase a,
when
muscle activity ceases or an animal recovers from fright. Many features
of the system for activating phosphorylase are shared with the
activation
system for glycogen synthesis, but the shared features are opposite in
effect. Thus, the myofibre does not attempt to synthesize
new glycogen
at the same time that it is breaking it down, and vice versa.
Newborn pigs often have difficulty maintaining their blood glucose
levels,
and may die of hypoglycaemia
after even short periods of starvation. Given they have high glycogen
levels at birth, this is not easy to explain,
although stored glycogen may simply be inadequate in amount if milk is
not available. Despite an adult predisposition to the excessive
accumulation
of adipose tissue, newborn pigs have very little adipose tissue and
liberation of free fatty acids is severely limited. Most phosphorylase
is in the relatively inactive b form just after birth. In adult pigs, hyperglycaemia
readily occurs in response to exercise and adrenaline secretion.
12. 6 Fate of lactate in living animals
Meat animals may exhibit a considerable range in their general muscular
and cardiovascular fitness. At one extreme, sheep and cattle may roam
in
search of feed and water for part of the year, coping with adverse
conditions
much like wild animals. At the other extreme, animals may be reared in
close confinement, partly to prevent them wasting feed energy by
converting
it to muscular work. Pigs rarely exercised may have a low oxygen
transport capacity whereas regular exercise reduces lactate production.
In living animals, the lactate produced by contracting muscles is
removed
by the circulatory system, but first it must travel from the myofibre
into the interstitial space. Lactate release may be retarded in
situations
such as exhaustive exercise. A fraction of the lactate leaving a
myofibre
may be in the form of undissociated
lactic acid, and this fraction may
increase with a rise in external pH. In living animals, blood flow is
increased
in active muscles (hyperaemia).
When hyperaemia occurs as a secondary response
to hypoxia, it may be mediated by the release of adenosine (from AMP A
+ P) into the interstitial fluid between myofibres.
On arriving in the liver, lactate may be converted to
glucose-6-phosphate,
and then stored as glycogen or released as glucose back into the blood.
Circulating glucose is available to muscles to be stored as glycogen or
used directly as energy for contraction (Cori cycle). After exhaustive
exercise, an animal may continue to consume oxygen at an increased rate
for some time (oxygen debt).
During this recovery period, some lactate
may be completely oxidized to release enough energy for the remaining
lactate
to be converted to glucose (gluconeogenesis)
in the liver. When an animal
is exsanguinated, the blood can no longer perform its transport
function
in the Cori cycle, and lactate accumulates in the musculature. There
are,
however, a number of other possible fates for lactate in living
animals.
Heart muscle receives its own blood supply from the coronary artery,
straight from the aorta, and cardiac muscle may use circulating
lactate
as an energy source. The high oxygen concentration in the aorta
usually
allows complete oxidation of lactate by cardiac muscle. Any lactate
remaining
in the aorta may not all get pumped to the liver or kidneys to
participate
in the Cori cycle, because the aorta supplies other major arteries in
addition
to the hepatic and renal arteries. Much of the circulating lactate may
pass through non-contracting muscles oxidizing lactate as an energy
substrate, particularly if circulating fatty acids are unavailable.
Resting
muscles also may take up circulating lactate and reconvert it directly
to glycogen, probably via a pathway which is independent of the
mitochondrial
Krebs' cycle (the evidence is in research papers even if not in your
biochemistry book). Finally, also it is possible for all the lactate
consumed
during an oxygen debt period to be oxidized to carbon dioxide.
Contraction causes an initial drop in myofibre pH because of the
hydrolysis of ATP, but this may be followed by an increase in pH from
the
breakdown of creatine phosphate
(CP). CP acts as a short term store of
energy since it has a phosphate transferable to ADP by the creatine phosphokinase (CPK). CP is
the dominant carrier
of energy from mitochondria to myofibrils. CPK and creatine are
normally
contained within myofibres, but may leak into the blood from damaged
or diseased myofibres. In healthy muscle, creatine is slowly but
continuously
converted to creatinine.
Creatinine is lost in the urine in approximate
proportion to the muscle mass of the body. The production of lactate
following
muscle contraction may cause another drop in pH. The interstitial pH
between
muscle fibres and the pH at the muscle surface are profoundly affected
by overall pH levels in the blood.
12.7 Effect of pH on water-holding
- Now we can see why the pH of meat generally declines after
slaughter
- the lactate accumulates as a byproduct of the process that releases
energy
in an attempt to keep the cell alive. And now we can explain how this
affects
the quality of the meat, firstly, its water-holding capacity.
- If dried meat protein is rehydrated by exposure to increasingly
damp air, three water compartments may be detected by the way in which
water is taken up: 4% of the water becomes firmly bound as a monolayer
round muscle proteins, another 4% is taken up as looser second layer,
and
10% of the water accumulates loosely between protein molecules.
- Water binding capacity is
the ability of meat to bind its own water
or, under the influence of external forces such as pressure and heat,
to
bind added water.
- Water absorption or
gelling capacity is the ability of
meat to absorb water spontaneously from an aqueous environment.
- Water binding
capacity and water absorption are closely related.
- Water absorption may
be found from the increase in weight and volume of meat samples placed
in an aqueous solution, and water binding capacity may be determined by
centrifugation or by pressing the fluid from the meat and measuring it.
- Water binding capacity is
modified by pH and drops from a high
around pH 10 to a low at the isoelectric
point of meat proteins between
pH 5.0 and 5.1.
- At its isoelectric point a protein bears no net charge
and its solubility is minimal.
- Below pH 5, a value only attained if the
pH of a processed meat product is deliberately lowered , water binding
capacity starts to increase again.
- Thus, as the pH of pork declines post mortem,
its water binding capacity decreases, and much of the water associated
with muscle proteins is free to leave the myofibre.
Further information
Structure and Development of Meat
Animals and Poultry. Pages 495-548.