11 Contraction
11.1 Introduction
Within each myofibre
are one or two thousand myofibrils - each capable of contraction by
filament sliding. Thick myofilaments move past thin myofilaments
and sarcomeres shorten - their Z-lines move closer together. To
explain a little of how this happens we need to introduce the following.
- Transverse tubule
- connects to the surface membrane of the myofibre.
- Sarcoplasm - the
cytoplasm around the myofibrils.
- Sarcoplasmic
reticulum - a membranous system within the sarcoplasm and around the
myofibrils.
- The sarcoplasmic
reticulum is activated by transverse tubules.
- The sarcoplasmic
reticulum stores calcium ions and releases them to trigger muscle
contraction.
- The sarcoplasmic
reticulum resequesters (grabs back) the calcium ions to allow
myofibrils to relax.
- Calcium ions are
detected by troponin - a protein on the thin myofilament.
- Troponin moves
tropomyosin - another protein on the thin myofilament.
- With tropomyosin
out of the way, myosin molecules on the thick myofilament grab and
swivel against actin molecules on the thin myofilament.
- Thick myofilaments
move past thin myofilaments and the myofibril contracts.
This information
will be essential later for understanding meat toughness. If you
cannot agree this is important - please drop this course and find
another career. We already have enough people producing tough meat.
11.2 Sarcoplasm
- The cytoplasm within a myofibre is called sarcoplasm.
- The aqueous phase of the sarcoplasm is called the cytosol.
- The calcium ion
concentration of myofibre cytosol is regulated by the sarcoplasmic
reticulum.
- The sarcoplasmic reticulum surrounds
the myofibrils within a myofibre and occupies about 4% of the
volume of
the myofibre.
- In a resting myofibre, the cytosol is kept almost free of
calcium ions by the rapid sequestering of calcium ions by the
sarcoplasmic reticulum.
- The concentration of calcium ions in a resting myofibre is
about 5 x 10-8
M. This is increased to
about 5 x 10-6
M when the myofibre contracts.
- The protein calsequestrin
binds and
stores calcium ions within the sarcoplasmic reticulum.
- Calsequestrin concentration is higher in fast‑contracting
than in
slow-contracting myofibres.
11.3 Sarcoplasmic reticulum and transverse tubules
The sarcoplasmic reticulum
initiates muscle contraction by releasing calcium ions when prompted to
do so
by the transverse tubular or T system.
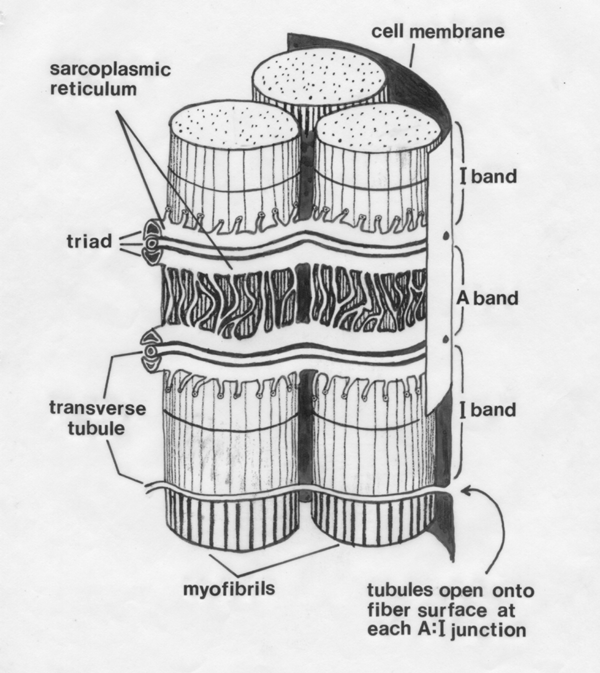
Each T tubule is
a finger‑like inpushing from the surface membrane of the myofibre, coming into very close contact with elements
of the sarcoplasmic reticulum.
- T tubules
conduct action potentials initiated at the neuromuscular junction and
carry them deep into the
interior of the myofibre.
- Communication between a transverse
tubule and the sarcoplasmic reticulum is via protein‑containing bridges between adjacent
membranes.
- The bridges are composed of four subunits.
- The bridge proteins extend through the membrane of the
sarcoplasmic reticulum to form calcium
ion channels.
- At the ends of muscle fibers where transverse tubules
may not reach, the sarcoplasmic reticulum may be directly connected to
the
plasma membrane.
- An extracellular marker, horseradish peroxidase, can
move into the transverse tubules, thus proving
they open onto the myofibre surface.
- In the
striated muscles of our meat animals, T tubules occur at the level of
each A‑I
junction.
- After contraction has occurred, it is turned off if
the sarcoplasmic reticulum resequesters all the calcium ions it
initially
released.
- Sustained muscle contraction or tetanus is the result of the fusion
of individual muscle twitches.
- The peak tension generated by a single twitch
occurs a few milliseconds after the action potential on the myofibre
membrane, when about 60% of the maximum calcium ion release has
occurred.
11.4 Steps of contraction
(1) The sequence of events
leading to contraction is initiated somewhere in the central nervous system,
either as voluntary activity from the brain or as reflex activity from
the
spinal cord.
(2) A motor neuron
in the
ventral horn of the spinal cord is activated, and an action potential passes
outwards in a ventral root of the spinal cord.
(3) The axon branches to supply
a number of myofibres called a motor
unit, and the action potential is conveyed
to a motor end plate on each
myofibre.
(4) At the motor end plate, the
action potential causes the release of quanta
(packets) of acetylcholine
into
the synaptic clefts on the
surface of the myofibre.
(5) Acetylcholine causes the
electrical resting potential
under the motor end plate to change, and this then
initiates an action potential that passes in both directions along the
surface
of the myofibre.
(6) At the opening of each
transverse tubule onto the myofibre surface, the action potential
spreads
inside the myofibre.
(7) At every point where a
transverse tubule touches part of the sarcoplasmic reticulum, it causes
the
sarcoplasmic reticulum to release calcium ions.
(8) The calcium ions are detected by troponin and
movement of tropomyosin on thin myofilaments
enables myosin molecule heads to "grab and swivel" their way
along thin myofilaments. This
is the driving force of muscle contraction.
Contraction is
turned off by the following sequence of
events.
(9) Acetylcholine
at the
neuromuscular junction is broken down by acetylcholinesterase, and this
terminates the stream of action potentials along the myofibre
surface.
(10) The sarcoplasmic reticulum
ceases to release calcium ions, and immediately starts to resequester all the
calcium ions released.
(11) In the absence of calcium
ions, there is a change in the configuration of troponin, and
tropomyosin then blocks the
action of the myosin molecule heads, and contraction ceases.
(12) In the living animal, an
external stretching force, such as gravity or an antagonistic muscle,
pulls the
muscle back to its original length. In other words, there is no special
system to increase the length of a muscle.
Muscle contraction may take either of two forms, or a
combination of
both. In isotonic contraction,
the muscle shortens against a constant
load
which is moved. In isometric
contraction, the load is too great to be moved,
and the muscle generates tension
as it attempts to shorten.
In slow‑contracting
myofibres the accumulation of calcium ions by mitochondria may
contribute to muscle relaxation.
11.5 Thick & thin myofilament structure
On the left of the diagram below we see how globular actin molecules
are arranged in a double helix to form thin myofilaments. On the
right is a single myosin molecule. In the middle is a thick
myofilament showing protruding myosin molecule heads.
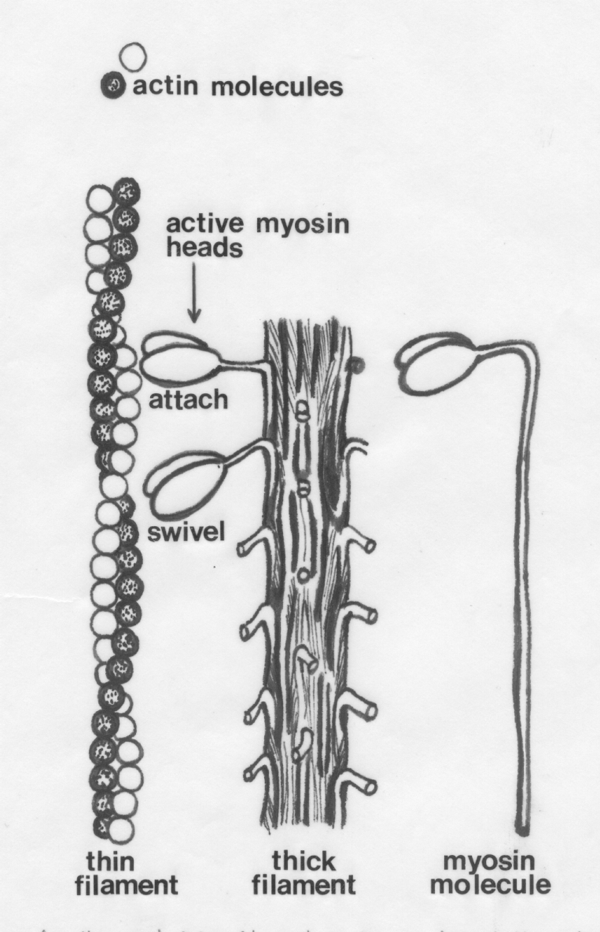
Troponin and tropomyosin on the
thin myofilament control the access of the myosin molecules to the
active sites on the actin molecules. Contraction is turned ON when the tropmyosin is IN the groove of the thin
myofilament. Contraction is turned OFF when tropomyosin is OUT of the groove.
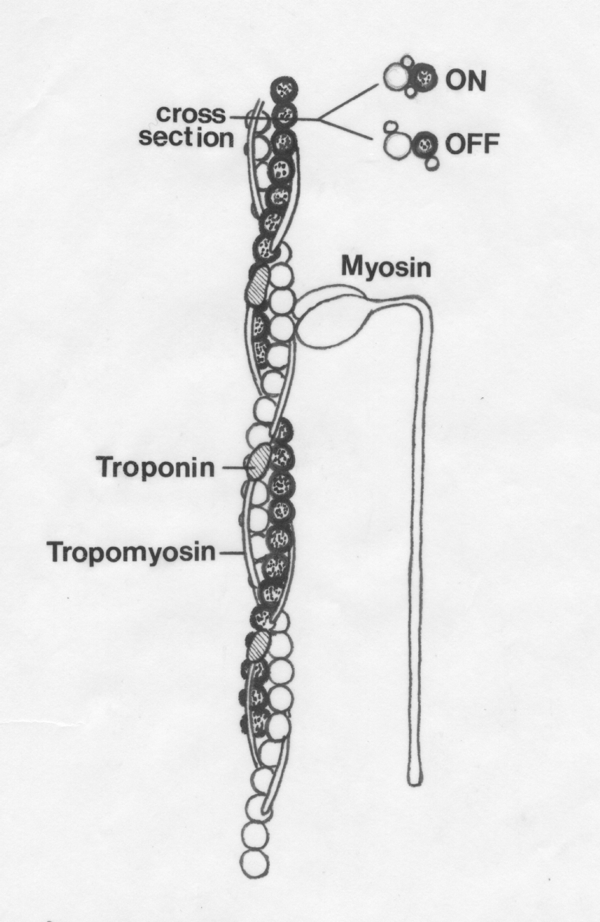
11.6 Myosin
- The proteins of the myofibril
account for somewhat more than half of the protein content of most
myofibres.
- Within the myofibril, myosin accounts for 55 to 60% of
the protein
content and actin accounts for about 20%.
- The
general shape of the myosin molecule is shown below.
-
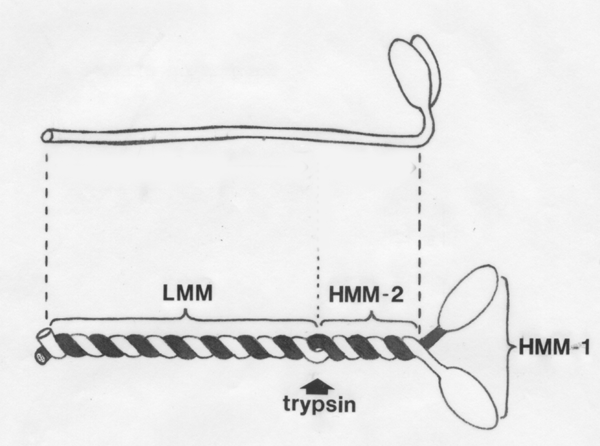
- Experimentally
isolated myosin molecules are just large enough to be seen by electron
microscopy after they have been sprayed with atoms of metal.
- The two active
heads of each molecule are difficult to resolve separately, and so the
molecule
usually has the appearance of a miniature matchstick with only one
globular
head.
- Myosin may be extracted in about 15 minutes with a
concentration of 0.5 M NaCl.
- Myosin is a
long
asymmetrical molecule composed of two
heavy chains (220 kDa)
and four light chains.
- Two of the light chains (LC2,
18kDa) have a regulatory
function and the other two are essential for ATPase activity.
-
The
digestive enzyme trypsin
splits the myosin molecule into two large fragments, (1) light meromyosin (LMM) with
a molecular weight of about 140 kDa, and (2) heavy meromyosin (HMM) with a weight
of
about 340 kDa.
-
When
light meromyosins are placed together under conditions
similar to those inside a myofibre, they self-assemble into a bundle
that
resembles the backbone of a thick myofilament.
-
Papain splits heavy meromyosin
into two subfragments.
Papain is an enzyme obtained from tropical melons and is sometimes used to
tenderize meat.
-
Subfragment
1 of heavy meromyosin (HMM-1) is composed of the two active
heads of the myosin molecule, and these retain their ability to bind to
actin
and hydrolyze ATP.
The way myosin
molecules are arranged in a thick filament is shown below.
Half of the molecules in a filament face towards one Z‑line of their
sarcomere,
while the remainder face towards the opposite Z‑line. Although the
heads
protrude at regular intervals along the thick filament, there is a
short
headless zone at the midlength of the filament where the light
meromyosins of
opposite sides overlap by their tails. There are several hundred myosin
molecules in a thick filament, and the length of a whole filament is
about 1.5
microns.
11.7 Actin
- Individual
molecules or monomers of actin have a globular shape and are called
G‑actin.
- The polymerization of G‑actin to form filamentous F‑actin
requires energy from
ATP, and the resulting ADP is bound into the filament.
- G‑actin molecules have a
diameter of about 5.5 nm.
- Two strands of F‑actin are twisted around each other
to form the thin filament and the cross‑over points are about 35.5 nm
apart.
- Each complete thin filament has a length of about one
micron
and contains 300 to 400 G‑actins.
- Thin
filament assembly by actin polymerization is controlled by several
proteins,
binding either to the fast-growing or barbed end (gelsolin, villin, and capZ),
or to the slow-growing pointed end of the new then filament (tropomodulin in
association with tropomyosin).
- The overall length of the thin
filament may be controlled by a
"ruler" of nebulin.
- Although G‑actin is globular,
it has an active site on its
surface where it reacts with myosin molecule heads
during muscle contraction
- When G‑actin is polymerized to F‑actin, the
active sites
all face towards the H zone
and away from the Z‑line in
which the thin filament
is anchored.
- Thus, within an individual sarcomere, the thin filaments
on either
side of the H zone are mirror images and are compatible with the the
arrangement of myosin molecules in the thick filament.
- If a thick filament
should penetrate the Z‑line, the immediate thin filaments in the next
sarcomere
are facing the wrong way for the myosin molecule heads of the intruding
thick
filament.
Further information
Structure
and Development of Meat Animals and Poultry. Chapter 5.